3D-printed microscopic cages confine bacteria in tiny zoos for the study of infections
October 10, 2013
[+]
By caging bacteria in microscopic houses, scientists at The University of Texas at Austin are studying how communities of bacteria, such as those found in the human gut and lungs, interact and develop infections.
The
researchers use a novel 3-D printing technology to build homes for
bacteria at a microscopic level. The resulting structures (imaged in red
through confocal fluorescence) can be of almost any shape or size, and
can be moved around in relationship to other structures containing
bacterial microcommunities (imaged in green). (Credit: Jason
Shear/University of Texas at Austin)
In a recent experiment, they demonstrated that a community of Staphylococcus aureus, which can cause some skin infections, became more resistant to antibiotics when it was contained within a larger community of Pseudomonas aeruginosa, a bacteria involved in various diseases, including cystic fibrosis.
The researchers use a novel 3-D printing technology to build homes for bacteria at a microscopic level. Their method uses a laser to construct protein “cages” around bacteria in gelatin.
The resulting structures can be of almost any shape or size, and can be moved around in relationship to other structures containing bacterial microcommunities.
The method should enable an entirely new class of experiments that better approximate the conditions that bacteria encounter in actual biological environments, such as those in the human body.
“It allows us to basically define every variable,” said Jodi Connell, a postdoctoral researcher in the College of Natural Sciences. “We can define the spatial features on a size scale that’s relevant to what a single bacterium feels and senses. We can also much more precisely simulate the kinds of complex bacterial ecologies that exist in actual infections, where there typically aren’t just one but multiple species of bacteria interacting with each other.”
Recipe for cooking and using a cage for bacteria
Here’s how Connell and her colleagues, including Jason Shear, professor of chemistry, and Marvin Whiteley, professor of molecular biosciences, do it:
- Identify which bacteria you want to cage and in what shape.
- Start with a gelatin-based reagent. Bacteria can live and reproduce comfortably within it. When warm it’s a liquid solution but at room temperature becomes firm like JELL-O. And it has within it photosensitive molecules that cause gelatin molecules to react and link together when struck by laser light.
- Put the bacteria in the solution and cool it, causing the bacteria to become fixed in place.
- Fire the laser (it uses a chip adapted from a digital movie projector to project a two-dimensional image into the gelatin). Wherever it focuses, a solid matrix forms.
- Do another layer, and another, and so on, building up.
- After the structure is completed, feed the bacteria nutrients and allow them to reproduce within a confined space to a controlled density.
- Optional: take other caged microcommunities and put them close enough together for the communities to signal to each other. Or wash away the excess gelatin, arrest the growth of the bacteria, and store them for later transport to labs in other parts of the world.
Bio-friendly
“What’s key is these structures aren’t just controllable in terms of their geometries; they’re also very bio-friendly,” said Shear. “The walls that we make out of these protein molecules are linked together tightly enough to prevent the bacteria from escaping, but they are porous enough to be chemically permissive. Nutrients can come in. Waste can go out. Signals can be exchanged. They’re trapped in these tiny houses, but they function like they do in biological environments.”
Shear said the new technique should enable a vast range of experiments. Growth can be arrested at any point to do gene expression analyses of bacteria, to see which genes are turned on or off in response to conditions. Multiple bacteria can be forced to interact in different configurations, at different densities, over varying timescales.
Bacteria such as Staph and Pseudomonas can be arranged in a “core-and-shell,” with a core of Staph surrounded by a shell of Pseudomonas, to see what happens when they’re both confronted by an unwelcome intruder.
Bacterial conversations
[+]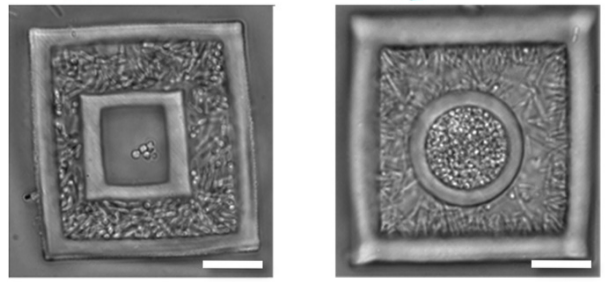
“These are really common bacteria that are often found together in
infections, and it makes sense that they would have mechanisms to sense
each other,” said Shear. “What the technology allows us to do is put
them in conversation with each other, in very precise ways, and see what
happens. In this case the Staph sensed the Pseudomonas, and one result was that it became more resistant to the antibiotics.”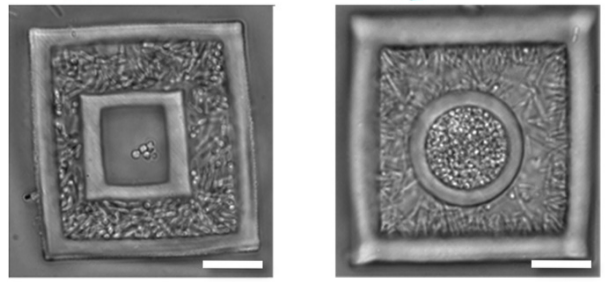
Engineering
polymicrobial communities. Low-density (Left) and high-density (Right)
S. aureus microclusters are confined in rectangular and hemispherical
cavities, respectively, surrounded by high-density P. aeruginosa
populations. (Credit: Jason Shear/University of Texas at Austin)
Among the long-term goals are to use the insights gleaned from such experiments to better combat infections in humans.
“Think about a hospital, which we know is not a good place to be to avoid infections,” said Shear. “There are studies that seem to indicate that infections are transmitted by very small microcolonies of bacteria, which are likely transported by equipment or staff from one part of the hospital to another.
“We currently know little about how this is happening. How many cells does it take? Do these microcommunities become particularly virulent or antibiotic resistant precisely because they’re small, and then in turn change the properties of bacteria on our skin or in our bodies? Now we have a means to start asking these questions much more broadly.”
When can we see these in use in hospitals and labs?
“The innovation here is a platform for conducting basic scientific studies into how polymicrobial communities may function, and specifically, how spatially dependent interactions between bacterial microclusters affects the virulence of microorganisms at infection sites,” Sheer told KurzweilAI.
“The time frame is now — we are already discovering differences between isolated bacteria/bacterial populations, and those organized within more complex communities. The technology builds on previous strategies for arranging bacteria in 3D within culture, and now allows one to organize essentially any set of geometries (e.g., nested colonies of desired shape) within walls that physically isolate distinct populations while maintaining chemical connectivity.
“This aspect is critical to our goals, as it allows free exchange across containing walls not only of nutrients and waste chemicals (necessary to enable colony growth) but also signals and ‘bio-warfare’ agents that bacteria use against each and against their multi-cellular hosts.”
Abstract of PNAS paper
Bacteria communicate via short-range physical and chemical signals, interactions known to mediate quorum sensing, sporulation, and other adaptive phenotypes. Although most in vitro studies examine bacterial properties averaged over large populations, the levels of key molecular determinants of bacterial fitness and pathogenicity (e.g., oxygen, quorum-sensing signals) may vary over micrometer scales within small, dense cellular aggregates believed to play key roles in disease transmission. A detailed understanding of how cell–cell interactions contribute to pathogenicity in natural, complex environments will require a new level of control in constructing more relevant cellular models for assessing bacterial phenotypes. Here, we describe a microscopic three-dimensional (3D) printing strategy that enables multiple populations of bacteria to be organized within essentially any 3D geometry, including adjacent, nested, and free-floating colonies. In this laser-based lithographic technique, microscopic containers are formed around selected bacteria suspended in gelatin via focal cross-linking of polypeptide molecules. After excess reagent is removed, trapped bacteria are localized within sealed cavities formed by the cross-linked gelatin, a highly porous material that supports rapid growth of fully enclosed cellular populations and readily transmits numerous biologically active species, including polypeptides, antibiotics, and quorum-sensing signals. Using this approach, we show that a picoliter-volume aggregate of Staphylococcus aureus can display substantial resistance to β-lactam antibiotics by enclosure within a shell composed of Pseudomonas aeruginosa.
(¯`*• Global Source and/or more resources at http://goo.gl/zvSV7 │ www.Future-Observatory.blogspot.com and on LinkeIn Group's "Becoming Aware of the Futures" at http://goo.gl/8qKBbK │ @SciCzar │ Point of Contact: www.linkedin.com/in/AndresAgostini